Minecraft, but it's biomining (!)
The multifaceted problems of an increasing demand in energy-transition metals, e-waste accumulation, and the multi-billion-dollar opportunity to solve them through biomining
Yeah, LK-99 was hot for a week or two. Itâs like Ron Weasley trying the Wingardium leviosa and getting it wrong⌠but you know thereâs something as huge and ancient as the Stonehenge stones, still burning AF, and could make or break all of your solarpunk dreams?
Now that I have your attention đâŚ
Your smartphone and laptop, the Tesla you wish you had enough money to buy or the Nissan you already have, MRIs and X-Rays for exceptional healthcare, electricity networks, military equipment for who knows what, photoluminescence, and the millions of solar panels, wind turbines, and nuclear reactors that the UN somehow expects the world to adopt by 2030 to reach our climate goalsâthey all depend on a kind of metals called Rare Earth Elements (REEs) + Lithium (Li), Iron (Fe), and steel (Fe-C).
And guess what! We are not nearly as close to meeting the demand for these in the next couple of years, let alone the demand according to the UNâs goals. Not only that, but mining is currently a very energy intensive and environmentally unfriendly industry, contributing with up to 8% of CO2 emissions, seriously polluting our water bodies, and posing very interesting geopoliticalâeven interplanetary đ°ď¸âquestions.
Sounds familiar? I found it ironic: we think of EVs and renewable energies as *the* solutions that will move us away from petroleum⌠but if we dig deeper into the problem, we find ourselves still digging holes in search for white and silver, as opposed to black gold1.
Is there any chance that technology even more ancient than this industry can satiate our cravings for metals sustainably, perhaps boosting a âneo-metallumâ era??? đ
The first âmetal-eatingâ microbes were isolated and characterized in the 1920s, biomining has been in use to extract uranium since the 60s, and in fact, over 15% of copper has been biomined annually since the 90s.
Wait but why?âyou ask.
The short version is that, at a low price and good enough specificity and yield, these microbes can eat the ores encapsulating our precious REEs. Although this has mostly been used in downstream processing of the ores, an ideal case would be to substitute the archaic use of explosives with in-situ biomining so we can get our preciousssss, just like Gollum.
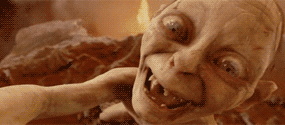
It is about time to show you, in 3 simple sections, a bit more of what Iâve learned:
The Solar Minecraft Map: resource-rich countries, Chinaâs leadership, and extending outside of Earth = the problem and opportunity.
How TF microbes eat metals: how mining currently works, an atom or two on the science of biomining and companies in the space = status quo and potential solutions.
Bottom line: the questions I did not get to answer here but youâyes, youâmay have the answer?
The Solar Minecraft Map
SO! If you want the long version, our friends at IEA have one of the most comprehensive reports I found. If you want the TLDR: production and processing of >75% of minerals and some REEs is concentrated with only 3 producers. Can you guess who those are?
It doesnât take a magnifying glass to notice how centralized these supply chains are in comparison to other commodities like oil and natural gas. Though this is especially true on the processing end, I would like to spotlight countries like Chile and the Democratic Republic of the Congo, and certainly China, that are home to a large portion of these metals.
Lithium
China does not only possess large quantities of Li. They have invested themselves in being the worldâs leaders in low-cost production of EVs, batteries, solar panels, and wind turbines; they are sealing (buying?) multi-million-dollar agreements with countries like Kenya, who are to get a $666 m data center in exchange for permission to explore and exploit REEs and other resources; they are even expanding their research in Antartica, maybe hoping that the Madrid Protocol that forbids mining in the region expires in 2048 (letâs make sure it doesnât!).
If we just focus on Li, the map looks like this:
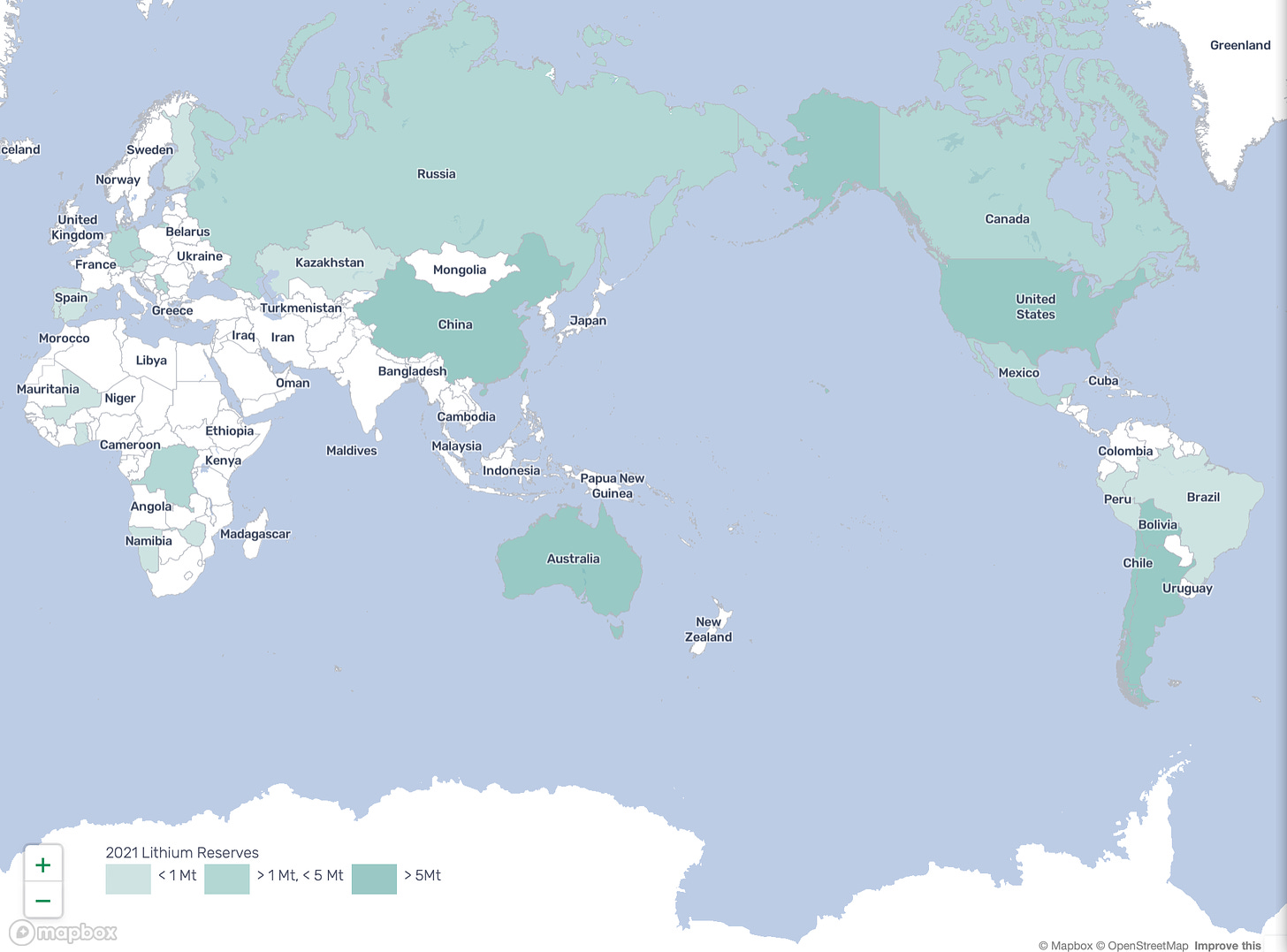
Bolivia, Chile, and Argentina form the âLithium Triangleâ. In Argentina, a 3% fee on the cost of the mineral extracted is charged to foreign companies for their operations, out of which provinces only receive 15% and the federal government stays with the remaining 85%. In Chile that amount can go up to 40%. Though these countries can also benefit by ensuring the creation of new jobs, nothing ensures the economic capital from these activities is not used corruptly, as has happened in Africa.
Conversely, Australia seeks lithium sovereignty, putting hundreds of millions of dollars into Li refining. âIf you have more of the supply chain in a country which has very strong governance, and a very, very safe and trustworthy business environment, then consumers can have more confidence in the products that they buyâ said Allison Britt, a director at Geoscience Australia, a government agency.
Exploration â data = unfair advantage
Now, if there is something that the mining and pharmaceutical industry have in common is that >50% of the time (>5 years) can be spent on exploration. This highlights the value of data on where these metals might be (da map!). The Kenyan government, for instance, withdrew $28.5 m in public funding only to map the countryâs mineral resources in favor of financing from China.
âChina will end up controlling the mining sector if they fund and carry out the survey because they will have raw data on the countryâs mineral wealthââKenya Chambers of Mines chief executive officer Moses Njeru.
Tools like AlphaFold and ESM have opened the doors to our protein multiverse, significantly shortening the drug discovery pipeline, providing a 10x better solution than crystallography. Similar AI innovations for faster REEs discovery will prove essential to meet the projected demand in the coming years.
Having lived in Monterrey for some timeâyes, the city in Mexico where the next Tesla GigaFactory is being builtâI got to know as much as the incentives to build the future in these cities as the social and environmental reasons not to do it: 65% of Li resources worldwide are located in areas of medium to very high water risk, and 98% of cobalt resources are found in areas of high social and governance risk:
Or perhaps, at some point, weâll have to reach theâŚ
Final frontier
I think that one of the awesomest characteristics of humankind goes beyond our cognitive ability to solve problems. It is the hope to imagine a more interesting future, coupled with the foolishness to actually create it (or at least die in the attempt of doing so).
The cost of space flight has decreased 10x since SpaceXâs Falcon 1, weâve now seen billionaires going to space themselves, and Musk claims they could get the cost of launching to only $2 m soon. To sum up, some debatable estimates point to 20-trillion-dollar opportunities in relatively small metallic asteroids of a 1.6 km diameter and there are millions of them out thereâŚ
However, and as much as I love the sci-fi-ness of all of this, the economics donât yet indicate that weâll be importing resources from space from now on⌠at least not in large amounts! Despite the billions of dollars that have been collectively spent on space mining since the 70s, these ships have so far only brought small samples (in the single-digit grams or less) for their inspection.
In 2020, Googleâs Planetary Resources Inc shut down. Although theyâd initially indicated that the platinum from a 30-meter-long asteroid could be worth $25â50 billion, they could have doomed themselves by rapidly increasing the demand for any metals outside of precious ones.
Rosa Santomartino, a postdoctorate researcher at the UK Centre for Astrobiology, offers a different perspective: âAs humanityâs presence in space plans to expand, we need to understand how to generate local resources and become independent from a constant resupply from Earth. The farther we travel, the more important this will becomeâ.
So it may not be until we actually need space manufacturing that it becomes economically feasible for the simple fact that weâre there. Cool that astronauts at the International Space Station (ISS) are already doing some trials though! Wanna learn how theyâre using microbes to mine Mars rocks?
How TF microbes âeat metalâ
The way itâs always been done
ALRIGHT! You could watch this 5-min cartoonish video or keep reading. Post-exploration, the actual mining, the drillinâ & sippinâ, comes in 3 different flavors (depending on the metals youâre craving):
Underground: deeper, more expensive, introduces chemicals that dissolve the target mineral, pokes holes on the ground and uses explosives to break apart the ores that surround the target. Used for gold, copper, lead, zinc, silver, nickel, lithium.
Surface: more shallow, less valuable deposits like coal, metals, gemstones, bauxite, sand, and limestone. Mainly used for coal, iron, and bauxite (aluminum), lithium.
In-situ: dissolving the mineral resource in place then processing it at the surface without moving rock from the ground. Primarily used for copper, uranium, and potash.
This provides useful information, not only in terms of optimizing the processes but also knowing what to optimize. If Li is mostly found at the surface, does that mean that itâs not and thus, despite its imminent increase in demand, new methods wonât make that much of a difference?
Even though this is not a rhetorical question (lmk!), I do know that 75% of energy in mining goes toward making steel, while most of the remaining ~25% is devoted to producing aluminum. Shoutout to my name-twin on her master-Metallurgy-piece for Contrary Capital!
Meet the microbes
So far, most biomining has been focused on pyrite-associated uranium, ferric iron, and sulfuric acid. This can either happen directly by changing the redox state of the target metal to make it more soluble, or indirectly by changing the redox chemistry of other metals that are coupled to the target.
Thiobacillus ferrooxidans (T. ferrooxidans or Ferro for us), is one of the most popular biomining tools out there. As you can appreciate in the following beauty (image), it obtains its energy from the oxidation of either ferrous iron or reduced sulfur compounds, making it useful to mine everything from copper to zinc, gold, uranium, nickel, and iron.
Much like plants, Ferro eats CO2 to get the carbon it needs to build its cell material and unlike mining man-made machines, it operates at relatively low temperatures which translates into lower energy consumption. In fact, the oxidation of sulfide minerals is an exothermal process which means that no heat is needed as an input.
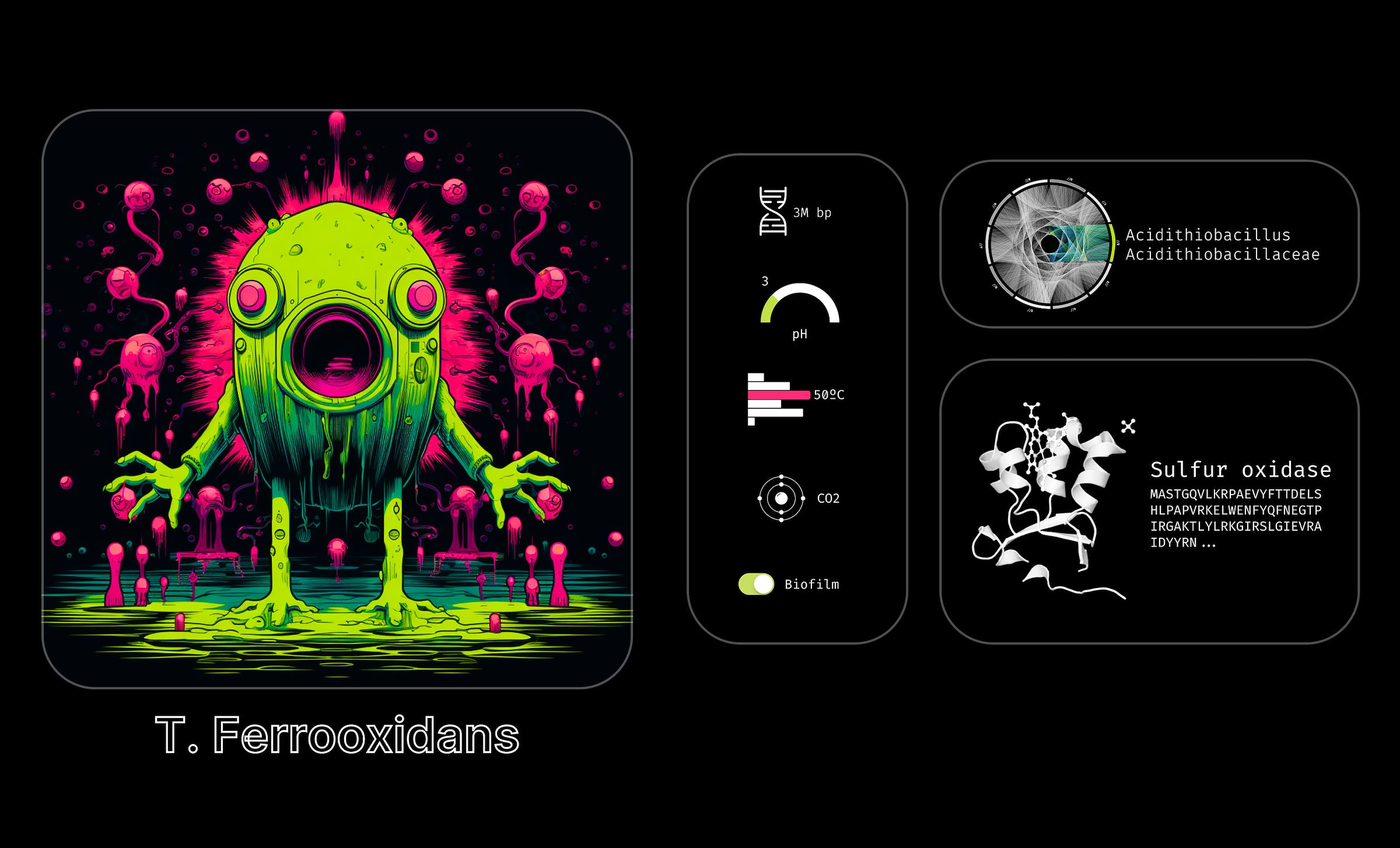
A little atom more of the science of this:
Sulfide minerals, such as pyrite (FeS2), chalcopyrite (CuFeS2), and arsenopyrite (FeAsS), contain metal ions like iron, copper, or arsenic that are bonded to sulfur
In an acidic environment, these minerals react with oxygen and protons (H+) to initiate oxidation reactions. These H+ ions facilitate oxidation by enhancing the reactivity of the species involved, thus promoting the breakdown of sulfide minerals
The oxidation of these sulfide minerals (catalyzed by microbes like Ferro) generates positively charged metal ions and sulfate ions (SO4^2-) as products:
As for the evolutionary history of these metal-eaters, I read here that before atmospheric molecular oxygen levels accumulated and permitted the existence of aerobic organisms; iron, arsenic, sulfur, manganese, other minerals and metals, were utilized in place of oxygen.
Something cool about bacteria is how collaborative they are. Through cell-to-cell contact, cells can chain, or link together, to pass electrons from one another and ultimately to the final electron acceptor, allowing all the cells linked in the chain to respire without the need for generating extra cellular machinery. You thought your $2,000 MacBook Pro 16âs transistors move electrons? Well, cells do too đź.
In the end though, mining is a matter of pH so I found the following diagram useful in illustrating how acidophiles maintain their pH: 1) potassium-transporting ATPases that are predominant in acidophilesâ genomes; 2) highly permeable cell membranes to decrease the influx of protons into the cell; 3) active proton export; 4) secondary transporters that reduce the energy required to pump nutrients into the cell; 5) enzymes/chemicals to bind protons; 6) DNA and protein repair systems; 7) idk.
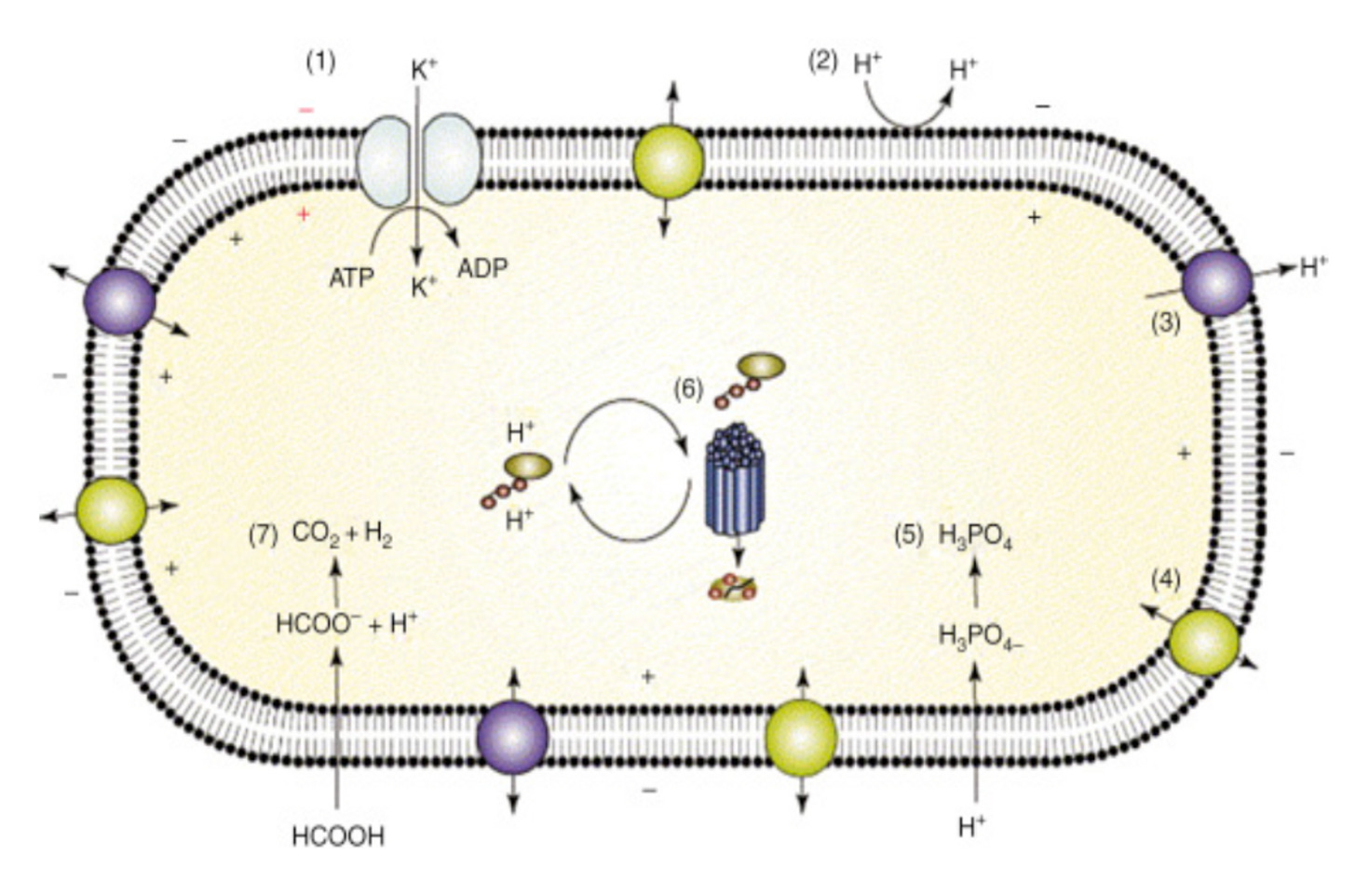
Importantly, not all microbes that eat ores can use that as their energy source. In fact, REEs are biomined from non-sulfidic ores that donât contain a usable energy source, so in that case, sugars need to be added to allow the microbes to grow.
Still, some non-obvious advantages of these biological tools are the easier recovery of metal by-products (such as silver) from processed ores than is the case with waste slags generated by pyrometallurgy as well as the fact that they can operate in a wide temperature spectrum. Just take a look at the catalog, I mean table of potential biomining tools:
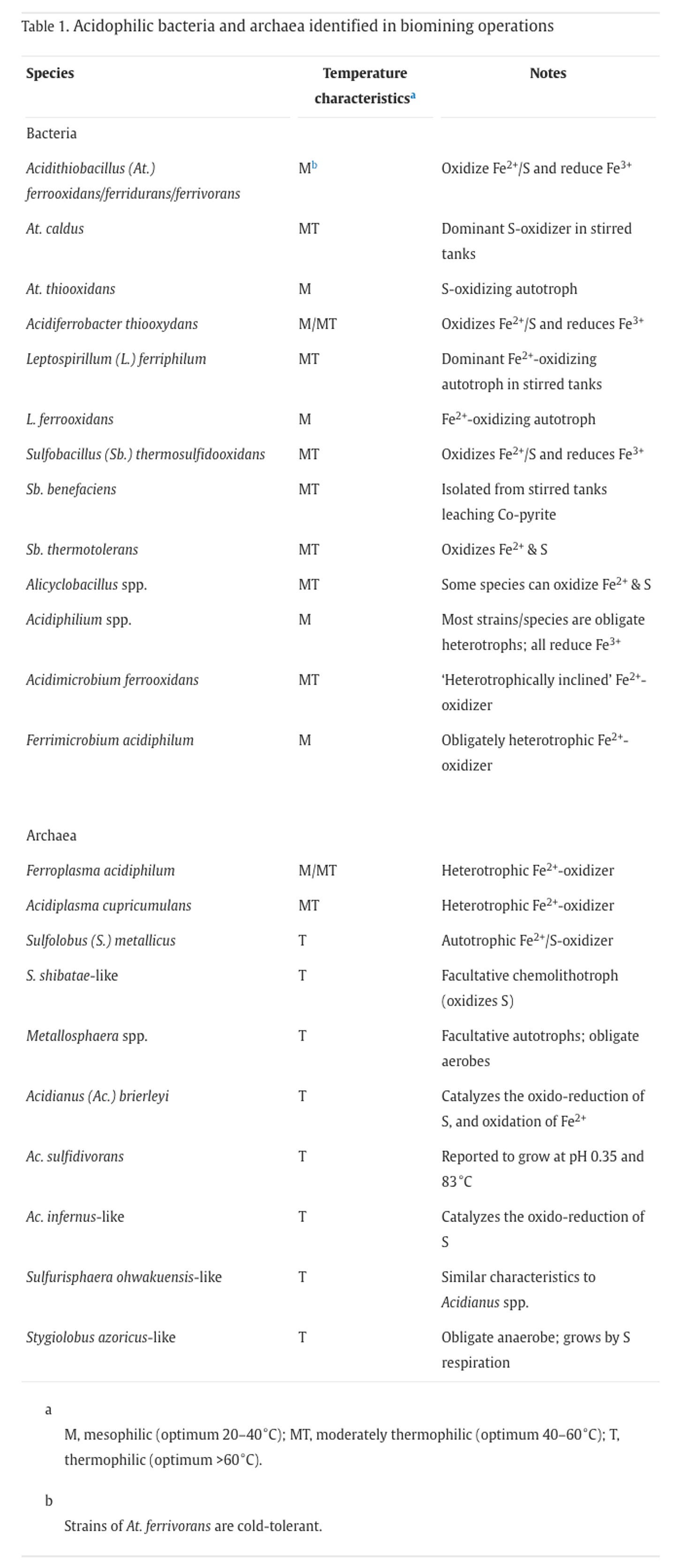
A grosso modo, the way these microbes are actually used as mining tools, can follow the in-situ mining process whereby we drill holes into promising ground and pump the microbes into the rocks which they then seep through to get their food. Once theyâre done, the minerals are extracted and the water is reused after the microbes regenerate.
Alternatively, and to prove that some version of this technology already scaled up long ago, the microbes can be used in huge (see image) tanks to process the ores that were collected through any other mining process.
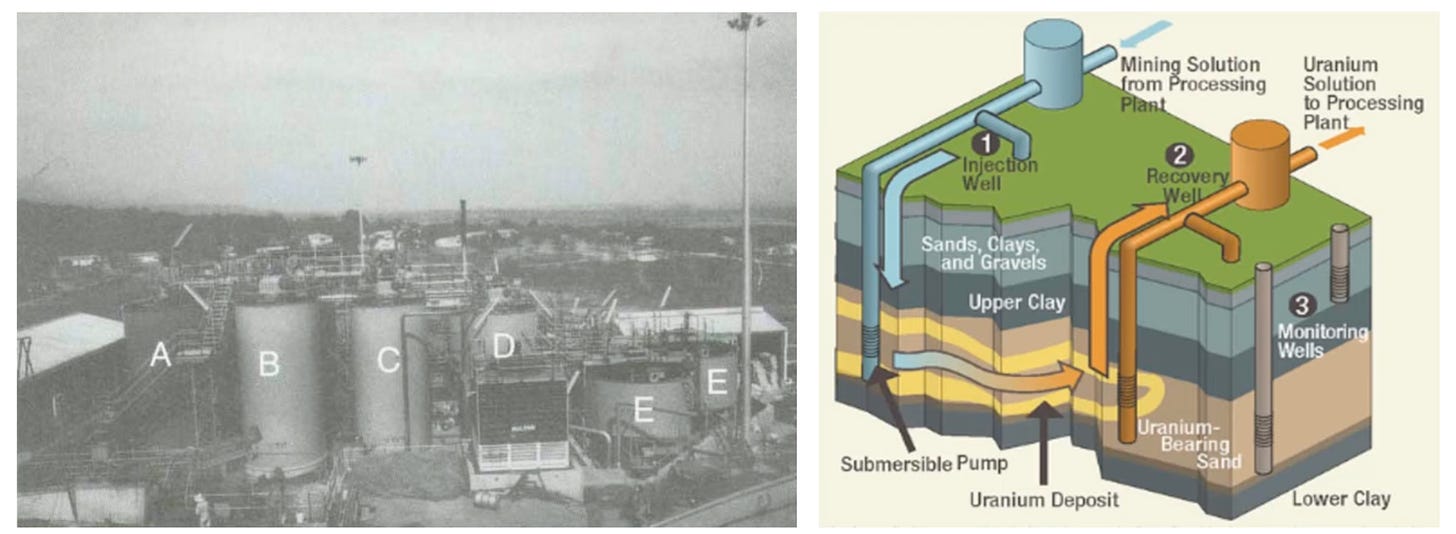
Solve E-Waste, solve demand
NOW. Thatâs kinda cool. But itâs time for my personal favorite part of the rabbit hole: E-Waste.
In her wonderful article, Anna-Sofia points out how âthe metal industry already has a pretty big circular economy goingâ as over 50% of the metal goods we use every day are made of recycled metals, and actually shoutout to her for including the following Periodic table of Recycled Content:
In analyzing this table though, we ought to consider: a) only 6% (3/50) of the elements shown do not have recycling rates higher than 50%; b) none of the elements that IEA estimates will have the highest increase in demand has high recycling rates; c) even though aluminum and steel, and copper are the main components in electronic devices like a smartphone or a laptop, thereâs still room for improvement in their recycling too; d) given the increasing demand, the economic incentives to recycle way more of the billion$ lost in e-waste are clear; e) e-waste has concentrations of gold 100s of times higher than gold ores.
On the not-so-bright side, the 53.6 million metric tonnes of e-waste produce nearly 100 Mt of CO2-equivalents (0.3% of global GHGs). Even when it is recycled, it exposes over 12.9 million informal waste workers to thousands of toxic substances.
This e-waste comprises small equipment (17.4 Mt), large equipment (13.1 Mt), and interestingly an increasing amount of temperature exchange equipment (10.8 Mt), especially in developing nationsâainât these climate feedback loops interesting? Screw climate â make ACs â screw climate with those too. Canât we just make sustainable things for our own sake, please?
The real root of the issue is that the elements in our e-devices are very similar to each other so separating them for recycling has thus far been time consuming, expensive, and dangerous. Ironically, processing 1 ton of REEs can produce 2,000 tons of more toxic wasteâdonât quote me on the law of conservation of matter here, idk where the Harvard magazine dudes got this fromâŚ
On the bright side, can you guess whoâs really good at separating specific metals? Oh yeah, the master of atoms baby, itâs biology baby! The Harvard research guys used Roseobacter sp. AzwK-3b, whose genus are strong metal consumers, as a filter of lots of metals called lanthanides. Their recipe goes like this:
Grow the microbes in artificial seawater at 37 ÂşC for ~2 months until getting a biomass of 0.05 mg/mL of medium
Immobilize 2 mL of the microbes on a 25 mm-diameter hydrophilic polypropylene filter with a pore size smaller than the diameter of the bacteria (0.2 and 0.8 Îźm respectively)
Pump 1 mL of the metal solution at 2.5 mL/min through the filter using a syringe
Wash with 5 mL of deionized water (pH 7) to remove any lanthanides not bound to the bacteria
Pump a series of 5 mL nitric acid solutionsâfrom pH 6 to 1.5, in intervals of 0.5 pH unitâpast the bacteria on the filter for desorption (liberation?) of the captured metals from the microbe
Other cool facts are that shooting the microbes with protons (fancy name: preprotonation) concentrates metals more than industrial processes can, and that light lanthanides desorb with higher-pH washes whereas heavy lanthanides desorb with lower-pH washes. Overall, the local maxima in the mass desorbed was at a low pH value.
Companies
BRAIN AG is a 30-year-old public German biotech that, among many other products across nutrition, health and environment, has built the BioXtractor: a mobile, fully equipped, self-contained pilot biomining plant for metal recovery from by-products, waste streams and primary resources.
Currently, they only use naturally occurring microbes to avoid GMO regulations and can make this process work in vats of 600 liters. They have at least 5 active patents on their technology.
Similarly, Mintbio has the vision of building âurban minesâ by having a precious metal biorefinery in every major city around the world. They grounds e-waste into a powder, add acids and oxidants to dissolve the metals into a liquid solution where the microbes are able to find gold and accumulate it on their surface. The gold-coated microbes can then be filtered out and refined to liberate the precious metal.
More recently, the startup Maverick Biometals graduated YC with the mission of mining Li and other metals in the most sustainable way possible. Through in-situ extraction, they use their proprietary LithXTMÂ enzyme combined in a water tank to degrade the ores and recover each metal selectively for commercial use.
Cool to see theyâre being advised by the legendary, the one and only, Esther Kim. In 8 months, the team developed their tech, took a polar expedition to the Artic -Circle to obtain microbe samples, visited major mining sites to better understand the mining sector and went through YC. What a group of shippers (miners? đˇ)
Another Texas-based young startup, EcoBiome Metals, has just conducted a successful 200-kg pilot study with BBX Minerals (public co) through their proprietary and patent-pending Gold DRIVETM tech to promote the recovery of 5E precious metals. BBX has initiated the necessary regulatory steps to enable the shipment of the microbes into Brazil, where theyâll conduct tests on an existing 13-tonne sample this year.
Similarly, in partnership with Arizona Lithium, Cemvita (Series A) has developed biomining tech to be used in heaps, tanks, or in-situ extraction of Li. Just at the start of last year, they opened a biofoundry in Denver to scale up the production of this and other products like hydrogen and carbon capture tech.
And the list goes onâŚ
Bottom line
Even though the microbes that these companies are used and have been using for decades have not been genetically modifiedâmaybe because of regulations or lack of scientific vision?âtheyâve been part of mining for decades. Now in this neo-metal era, I see biomining as one of the most interesting applications of synthetic biology given the economic opportunity, clear inherent benefits of the technology, as well as the few ethical questions of the technology itself compared to other applications.
Some questions that I still have though include:
Whatâs the cost of producing whatever amount of microbes to mine 1 ton of metal? How does that change the net cost of mining that ton of metal?
Is in-situ biomining a longer process because of the time that microbes take to metabolize these things?
What are some ways in which we can bioengineer better biomining microbes?
For now, I hope youâve enjoyed this article. Youâd make my day if you SHARE IT! Retweet, repost, restack, send it to your colleagues and friends and family, tag someone, etc :)
Additionally, if you think I should correct something, lmk. Iâm totally open to feedback but donât promise Iâll implement it fast these days.
Also, feel free to check out more of my posts (theyâre all shorter than this one, promise!).
Stay hard, see ya! âď¸đި ;)
It is important to clarify that despite the miningâs industry environmental impact, EVs end up having a lower net environmental impact than their counterparts.